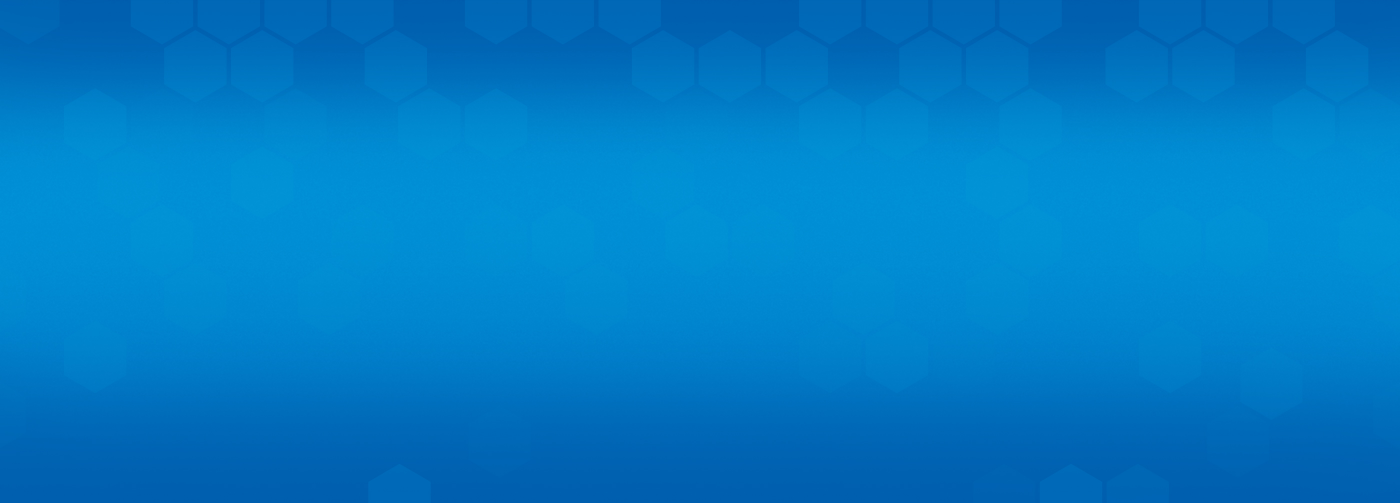
The classical approach to FRET measurements involves changing filter cubes. For example, in the acceptor-photobleaching method, a donor-specific cube is first used to collect the emission from the donor (e.g., CFP). Then a filter cube for the acceptor is used to visualize and photobleach the acceptor (e.g., YFP). Intensity measurements of the donor before and then again after photobleaching the acceptor are used to calculate FRET efficiency. Steep spectral edges of the filters ensure that only the acceptor is photobleached and minimize the signal contamination due to bleedthrough in multiply labeled FRET samples. This technique suffers from several drawbacks, including: slow speed (changing filter cubes takes typically a second or more) and imaging artifacts (due to the movement of the filter turret and other vibrations).
The most popular approach to FRET imaging, shown in Figure (b), is often called the FRET-cube method. A single-band exciter and a single-edge dichroic beamsplitter, each specific to the donor, are placed in a cube in the microscope turret, and a filter wheel with single-band emission filters is used to select the emission from either the donor or the acceptor. Filter wheels cause minimal vibrations and have much faster switching times (as low as 10’s of ms) compared to filter turrets, and are therefore better suited for live-cell FRET applications.
Many researchers prefer to utilize a Sedat filter set configuration. This approach provides additional flexibility in the visualization of the sample as well as to perform control experiments – such as finding regions or samples labeled with only the donor or acceptor and collection of pure spectral contributions from each. The added flexibility also enables the donor photobleaching method for calculation of FRET efficiency.
However, the most demanding FRET applications, such as live-cell imaging and imaging of single molecules may require “simultaneous” imaging of the emission signal from both the donor and the acceptor. Figure (c) shows a configuration for simultaneous imaging, in which an image-splitting dichroic beamsplitter placed in the emission channel of the microscope is used to separate the signals from the donor and the acceptor and steer them onto two different CCDs or two distinct regions of the same CCD. Since there are no moving parts, motion-based imaging artifacts are also eliminated.