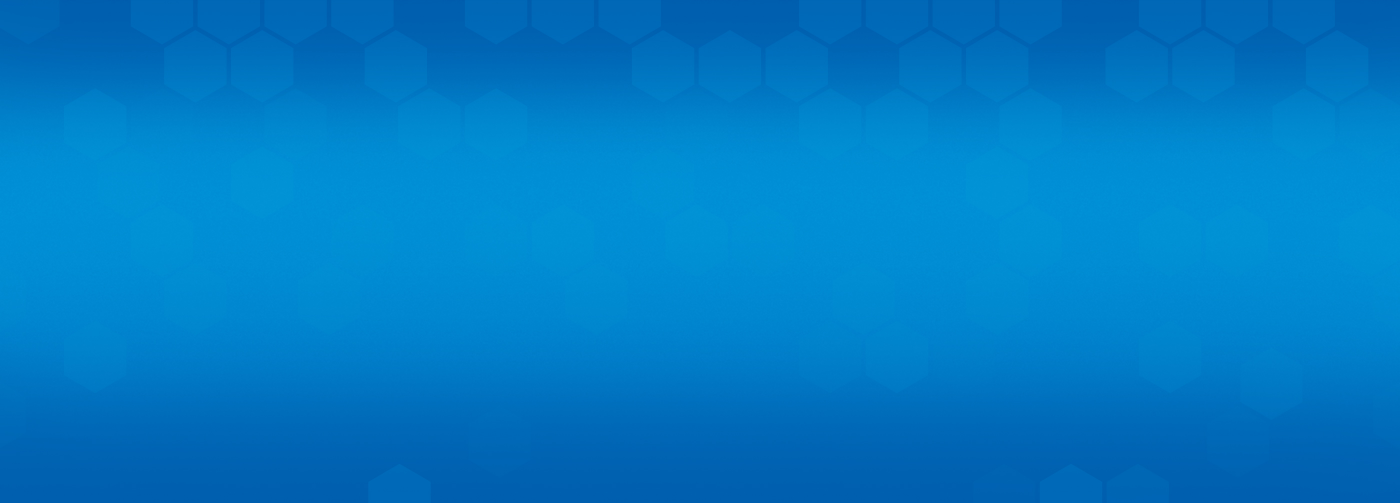
Life Science Optics
Cancer is a leading cause of illness and death for millions of people worldwide. The development of new medicines, treatments, and technologies to combat this disease is urgently needed. In this regard, optically based technologies are playing an increasingly important role in the detection, diagnosis, and surgical treatment of a large variety of cancers.
Standard surgical microscopy utilizes white light imaging to visualize cancerous tumors but poorly predicts tumor margins and may not be sensitive enough to detect small tumors. New developments, however, have merged fluorescence imaging with conventional imaging modalities. This new approach is commonly known as fluorescence guided surgery (FGS) or fluorescence image-guided surgery (FIGS) and can be employed in either open-field or minimally invasive endomicroscopy procedures. FGS uses fluorescent contrast agents administered preoperatively, followed by illumination of the surgical field during tumor resection. Fluorescence emission is specific to stained tumor cells, and detection of that emission allows improved tissue identification based on molecular differences. The major advantage provided by fluorescence imaging is therefore to provide the surgeon with a clear, high-contrast view that improves identification and delineation of tumor masses during surgery. It gives surgeons superior cancer-site navigation, safer resection, and higher sensitivity when compared to preoperative imaging, visual inspection with the naked eye, and palpation during surgery.
The success of FGS systems extends beyond tumor imaging and resection to a wide variety of clinical applications such as sentinel lymph node mapping, angiography, lymphography, and ureter and bile duct anatomical imaging. FGS can be used to preserve healthy cells and tissue during surgery, such as nerves and blood vessels, using special fluorescent tags which highlight these structures. FGS can also assist with imaging blood flow and tissue perfusion, identifying regions of poor blood flow that may lead to post-surgical complications.
FGS optical systems are designed with the same goal as that of non-surgical fluorescence imagers: to support high-contrast and high-resolution imaging that allows discrimination between fluorescently labeled and unlabeled tissues. However, clinical compatibility imposes additional performance requirements as compared to closed-field ex vivo imaging. While illumination should be limited to a narrow spectral band at the fluorophore excitation wavelength to minimize background signals and noise, white light illumination provides optimal reference for the surgeons trained to rely on visual information to identify anatomical landmarks and tissues in their native color. This requires viewing both the white light and fluorescence images on a single overlaid display, in real time and with co-registration, so the surgical team does not have to alternate between viewing the fluorescent imager display and the surgical field. Another desirable feature is fluorescence-mode operation under ambient uncontrolled light, since open-field FGS procedures are typically performed under bright white light illumination of a surgical room [1]. Given the comparatively weak intensity of the emitted fluorescence signals, achieving real-time fluorescence imaging together along with the overlay functionality imposes challenges on the system design, which can however be increasingly overcome in modern implementations.
Central to the success of the FGS approach is the utilization of non-toxic contrast agents. Currently, indocyanine green (ICG) is one of the most frequently employed NIR fluorophores used for FGS, though a few other contrast agents are clinically approved, including methylene blue (MB), 5-Aminolevulinic acid (5-ALA), and fluorescein sodium [2]. However, numerous fluorophores are commercially available, and many novel FGS contrast agents are under development [3] [4]. These new fluorescent agents are expected to support a paradigm shift in how molecular information is provided for surgical decisions.
Biomedical fluorescence imaging operates at visible wavelengths extending into the near infrared (NIR) spectrum. The majority of fluorescent probes emit light in the visible range (∼400-650 nm), which is not optimal due to accompanying tissue autofluorescence and high absorbance of visible light that limits the penetration depth to a few millimeters. NIR fluorophores are better suited for in vivo imaging and have excellent potential for FGS. The “NIR-I window” from 700 to 900 nm allows for deeper tissue imaging and enhanced sensitivity as the low tissue autofluorescence and reduced light scattering at NIR wavelengths further simplifies the task of filtering out background signals. The second near-infrared wavelength window (NIR-II, 1000 to 1700 nm) could further improve image-guided surgery [5]. However, few NIR-II probes currently allow dynamic imaging, and those available require use of exotic camera technology.
Fluorophore | Typical Peak Absorption / Emission (nm) | Filter Options |
---|---|---|
Fluorescein | 490 / 520 | FITC-2024B or LED-FITC-A |
PpIX from 5-Aminolevulinic Acid (5-ALA) | ~ 400 / ~ 630 | LD01-405/10 or FF01-389/38, with FF01-625/26 |
Cy5 | 650 / 670-680 | Cy5-4040C or LED-Cy5-A |
Methylene Blue (MB) | 670 / 690 | Cy5.5-C |
Cy5.5 / AlexaFluor 680 | 683 / 703 | Cy5.5-C |
Cy7 / AlexaFluor 750 | 750 / 775 | Cy7-B or LED-Cy7-A |
Indocyanine Green (ICG) | 790 / 820 | ICG-B |
IRdye® 800CW | 785 / 810 | IRDye800-33LP-A |
Table 1: List of commonly used fluorescent probes in FGS and filter options for instrument prototyping. Optimum filter choice depends on the overall system design, especially on the excitation source.
High detection sensitivity is one of the key performance requirements in FGS imaging. This high sensitivity allows the imaging system to display useable images that are distinguished from the biological tissue background even at low concentrations of fluorophores, such as with very small tumors in surgical resection. This sensitivity improves patient safety, diagnostic accuracy, and surgical outcome. However, imaging the weak fluorescence emission is challenging, and requires maximizing the signal-to-background ratio to allow distinguishing between diseased versus disease-free tissue, or identifying important structures to preserve, such as nerves, blood vessels, ureters, and bile ducts.
Achieving high signal and low background in a surgical environment is complicated in open-field surgery due to the high level of ambient lighting. Several factors influence the efficiency of fluorescence detection, and all components in the imaging system therefore require careful design and optimization. Among these, the optical filters used for excitation light sources, beam combination and separation dichroics, and emitted light are critical in maximizing detection sensitivity by limiting background light. Efficient spectral filtering reduces undesirable signals such as excitation or ambient light leakage while improving fluorescent signal collection.
Semrock is at the forefront of developments in fluorescence-image guided surgery. Due to the critical nature of FGS it is essential to use optical filters that offer the maximum brightness and contrast. In addition to off-the-shelf filters, we can help customize filters to achieve the best fluorescence intraoperative visualization, with maximum sensitivity and contrast resolution. Benefits of customized filters include:
Several companies across the USA and Europe have placed their trust in Semrock-brand filters to develop and deliver the right filters for their FGS instrumentation as they enter clinical trials.
Contact us today to discuss your custom optical filter needs
References
[1] DSouza AV, Lin H, Henderson ER, Samkoe KS, Pogue BW. Review of fluorescence guided surgery systems: identification of key performance capabilities beyond indocyanine green imaging. J Biomed Opt. 2016;21(8):80901. doi:10.1117/1.JBO.21.8.080901.
[2] Pogue BW, Rosenthal EL. Review of successful pathways for regulatory approvals in open-field fluorescence-guided surgery. J Biomed Opt. 2021;26(3):030901. doi:10.1117/1.JBO.26.3.030901.
[3] Nagaya T, Nakamura YA, Choyke PL, Kobayashi H. Fluorescence-Guided Surgery. Front Oncol. 2017;7:314. Published 2017 Dec 22. doi:10.3389/fonc.2017.00314.
[4] Barth CW, Gibbs SL. Fluorescence Image-Guided Surgery - a Perspective on Contrast Agent Development. Proc SPIE Int Soc Opt Eng. 2020;11222:112220J. doi:10.1117/12.2545292.
[5] Cao J., Zhu B., Zheng K., He S., Meng L., Song J., Yang H. Recent progress in NIR-II contrast agent for biological imaging. Front. Bioeng. Biotechnol. 2020
Imaging highlights tumor-free margins in cancer surgery
Nguyen QT, Olson ES, Aguilera TA, Jiang T, Scadeng M, Ellies LG, and Tsien RY, Surgery with molecular fluorescence imaging using activatable cell-penetrating peptides decreases residual cancer and improves survival, PNAS, 107, (2010), 4317.
Nguyen QT, Tsien RY. Fluorescence-guided surgery with live molecular navigation--a new cutting edge. Nat Rev Cancer. 2013;13(9):653-662. doi:10.1038/nrc3566.
Sajedi S, Sabet H, Choi HS. Intraoperative biophotonic imaging systems for image-guided interventions. Nanophotonics. 2019;8(1):99-116. doi:10.1515/nanoph-2018-0134.
Ruiz A J, Wu M, LaRochelle E P M, Gorpas D, Ntziachristos V, Pfefer T J and Pogue B W 2020, Indocyanine green matching phantom for fluorescence-guided surgery imaging system characterization and performance assessment, J. of Biomedical Optics, 25(5), 056003 (2020).